Advanced Turbines
Developing advanced turbine technologies in pursuit of power generation with higher efficiency, lower costs and lower emissions
The NETL Advanced Turbines Program manages a research, development, and demonstration (RD&D) portfolio designed to enable a carbon-free electricity sector and promote environmental justice by developing revolutionary, near-zero-emission advanced turbines technologies. In response to the nation’s increasing power supply challenges, NETL is researching next-generation turbine technology with the goal of producing reliable, affordable, diverse and environmentally friendly energy supplies. NETL is also committed to enabling the use of hydrogen in gas turbines to facilitate a future carbon-free electric power industry. Program and project emphasis is on understanding the underlying factors affecting combustion, aerodynamics/heat transfer, and materials for advanced turbines and turbine-based power cycles.
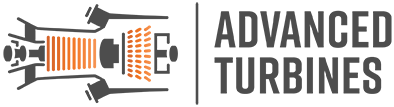
Explore Key Technology Areas
Advanced Combustion Turbines
Research addresses component development for turbine systems that are powered by various fuels, including hydrogen and natural gas in both simple and combined cycle applications. Topic areas include improved combustor designs to reduce nitrogen oxide NOx emissions, novel cooling schemes and thermal barrier coatings to protect first-stage turbine blades from higher turbine inlet temperatures, sensor development and aerothermal studies to enhance overall thermal efficiency.
Supercritical CO2 Power Systems
Research is focused on developing high-efficiency, low-cost power generation systems based on supercritical carbon dioxide (sCO2)-based power cycles. This includes new turbine systems for sCO2-based power cycles and development and testing of a sCO2 power cycle-based pilot plant.
Pressure Gain Combustion
Current research assesses the potential benefit of pressure gain combustion systems when used with gas turbines in both simple and combined cycles. Researchers are focused on combustion control strategies and fundamental understanding of pressure wave-flame interaction and lab-scale testing/component prototyping for integration with gas turbine engines.
Explore the Site
NETL implements this effort as part of the Carbon Management Technologies Program